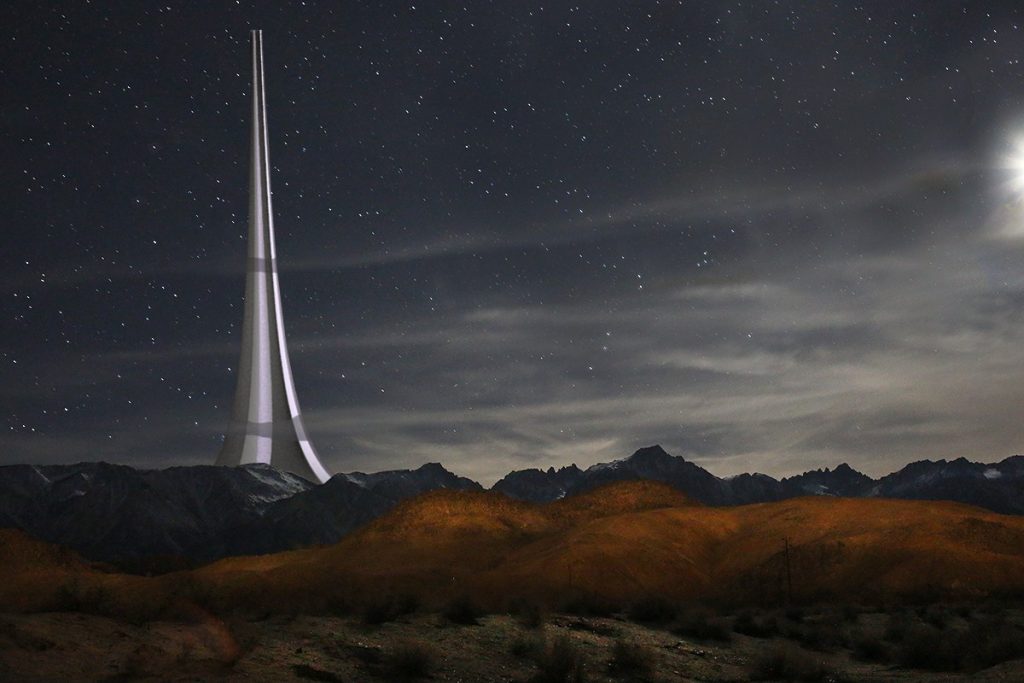
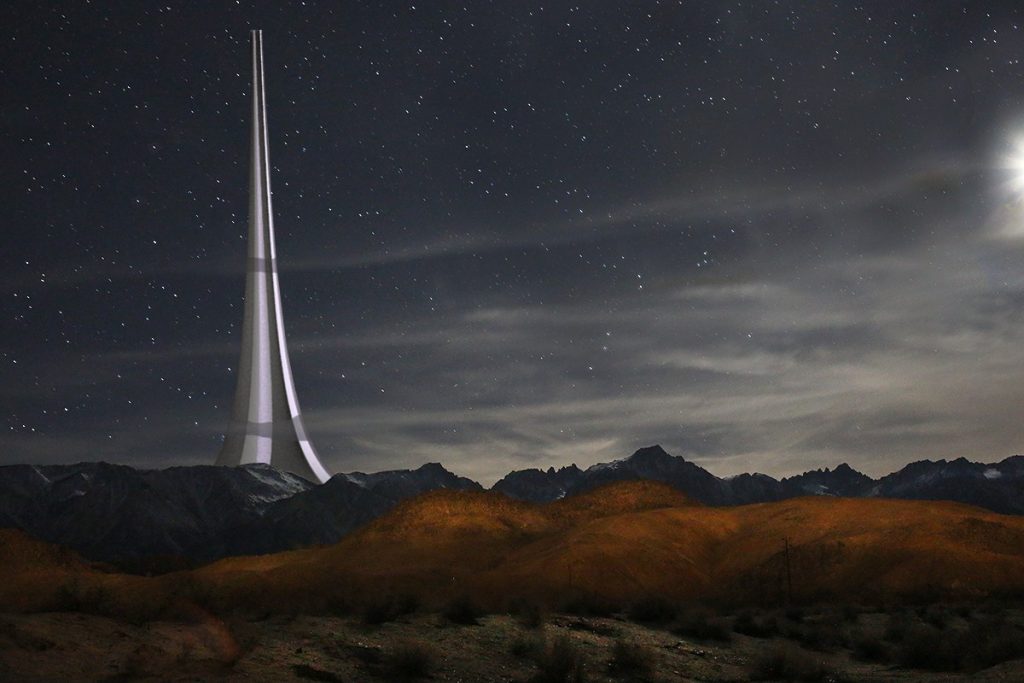
By Eric Domeier
Homo sapiens are a curious, exploring, conquering species. Our DNA is marked with this trait as strongly as it is with our ability to walk upright. No other species shares it.
About 10,000 years ago, a man or woman carved out a tree in what is now known as the Netherlands. They pushed that hollowed trunk into the water and took the first paddle towards trans-oceanic exploration. Known as the Pesse canoe, it probably did not go more than 100 yards before capsizing. But the first step was made.
When Burt Rutan stands next to SpaceShipOne, the first private manned spacecraft, he is little more than our first canoe maker. Currently one of the most successful designers, he will nonetheless be considered a primal ancient to the future sapiens of space.
Space is actually not that far away. The Karman line, the county line for space, is about the distance from San Diego to Los Angeles. But in getting to that 100 kilometer destination, one must overcome gravity, air resistance, and the Coriolis force (try to draw a straight line from the center of a rotating album; your line is going to end up being a spiral).


Space technology currently overcomes each of these forces with massive rocket power – massive, inefficient and explosive rocket power. Imagine a cruise liner buried in Robb Field at Ocean Beach. Now imagine strapping a rocket to the stern of that ship and blasting its furrowed way down Point Loma Boulevard, across Dog Beach, and out into the water until it gets deep enough to float. That’s more or less the efficiency of our current space technology.
So the engineers make smaller ships that don’t drag on the beach quite as hard. Smaller ships with smaller cargos. But, as with all exploration efforts, the primary goal is to get stuff — space labs, computers, food and people — out to our undeveloped destination. All of this weighs down the ship and increases the need for rocket fuel. According to NASA, it currently costs about $10,000 per pound of cargo to get to space. Assuming I’m not lying about my weight, my person is a $1.9 million payload. That’s a cost-prohibitive adventure.
A more efficient method for getting this stuff to space is with a tower. Discounting Babel’s Old Testament project, the space tower has been under discussion since the late 1800s. The advantage of the tower is to provide a mechanical means to reach a higher altitude. Basically an elevator. Where a rocket moves goods to space once every six months, the elevator is a virtually constant transfer. And safer and cheaper. Would you rather take a rocket backpack to your luncheon in the Symphony Towers or the elevator?
The possibility of the space tower has been approached primarily by physicists and rocket engineers whose expertise are not necessarily in the building sciences. It is now being explored by building designers. And the problem to be solved is fundamentally a structural one.
Implementing the 1:3 ratio of width to height for maximum stability, the base of our 100 kilometer tower will be 33 kilometer, which is about 20 miles wide. To reduce weight, the tower will be built with a space-frame structure. Of such massive scale, the structure will be exposed to dynamic and constantly shifting forces. These dynamic forces include ground settlement, expansion/contraction resulting from heat/cool cycles, wind and live loads (like the hoisting of a space craft). If the structure is too rigid, the structure will tear itself apart.
To accommodate these stresses, new space-frame technology will be developed. The structural system will include hydraulically adjusted members tied into a sophisticated sensor system. The structural frame will expand and contract in minute amounts across the vast planes of the structure to accommodate dynamic stresses. Additionally, the material will be built of monel or another impervious metal so as to avoid the need for additional coatings (paint) and reduce maintenance cost. Another advantage of the space-frame is that whole sections of it can be compromised (by something like a small asteroid) and the overall structural integrity will remain intact until repairs are made.
In context to the Celestial Tower’s 100-kilometer height, airliners travel at 12 kilometers, the atmosphere thins at about 80 kilometers, and the International Space Station orbits at 400 kilometers. Satellites find geosynchronous orbit at 36,000 kilometers.
What has been gained by providing a platform at 100 kilometers above sea level? Gravity at the top of the Celestial Tower is about 97 percent of what it is at sea level. So gravity remains mostly in full-force. But the top of the tower is above the thickest part of the atmosphere. As a result, atmospheric drag has been eliminated making propulsion significantly more efficient. Less fuel required to get to orbit and beyond. The tower may also become a platform for something like the Thoth Technologies Space Elevator (refer to The Guardian’s 2015 article “Going up? Space Elevator Could Zoom Astronauts into Earth’s Stratosphere.”
The cost of this project is roughly equivalent to 20 years of war. Maybe if we had something to strive for other than dominant control over diminishing global resources, war could be set aside. And to sate the appetite of the defense contractors’ hunger for wealth, let’s turn them on to the possibility of conquering Mars from the top of a Celestial Tower. After all, we are explorers first and warriors second. So it’s just a subtle shift in paradigm. And when my daughters take the elevator up, they can set their sights on new adventures and new resources. They will be the next generation of ancient heroes.
—Eric Domeier vive en North Park y practica arquitectura desde su oficina en Grim Avenue. Visite su sitio web en dome-arch.com o llámalo al 619-531-0010.